Preface
I gave this talk to my Honors Research Writing class at Walla Walla College in December 2003.
Since only my figures and a hazy outline survive, the following is a reconstruction of that
talk (with additions and improvements).
1. Introduction
I will attempt to explain all of Biology in the next 20 minutes.
2. Scientific Hierarchy
One way of looking at Biology is to put it in a hierarchy of subjects like so:
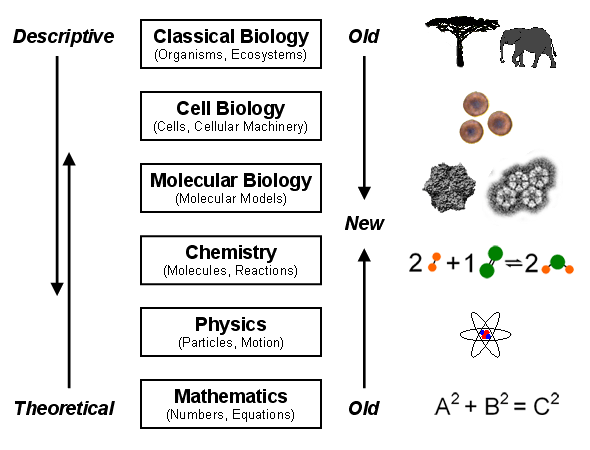
Historically, we have been working both down this hierarchy from the top and up this
hierarchy from the bottom.
People have been describing and classifying plants and animals for thousands of years
because all it requires is a pair of eyes. The invention of the light microscope brought
the discovery of cells and the beginning of Cell Biology. The quest to see things on a
smaller and smaller scale called for the invention of the electron microscope, which
revealed the inner workings of cells down to the molecular level.
People have also been playing with numbers and equations for thousands of years because
it only requires ten fingers and a stick to write in the dirt. Only recently did people
begin to use Mathematics as a background to describe physical phenomena. Sir Isaac
Newton invented Calculus to describe the physics of moving objects. As Physics grew,
atomic theory developed, which formed a nice basis for Chemistry. The focus of Chemistry
is how atoms form molecules. This led to Molecular Biology, which focuses on DNA and
Proteins - the large molecules found in living things.
The theoretical progression up from Mathematics and the descriptive progression down
from Classical Biology have met only recently, in the field of Molecular Biology.
This is very exciting because you can form a theoretical model of a large molecule,
and then see if your model is correct with an electron microscope. The
scale of Biology is now complete - all the way from individual atoms to populations
of organisms.
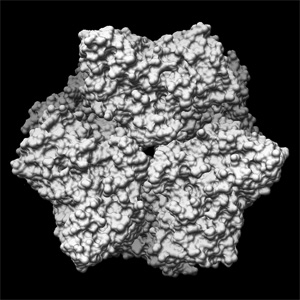 |
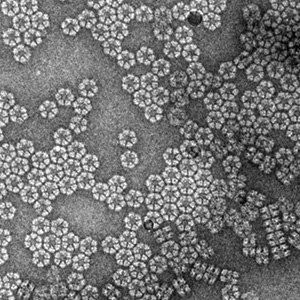 |
A biophysical model of a single hemocyanin hexamer. Hemocyanin
carries oxygen in the blood of invertebrates like crabs, similar to our hemoglobin. |
A picture of many hemocyanin hexamers taken
with an electron microscope.
Image courtesy of Dr. Sacha De Carlo |
3. Two Types of Experiments: functional and correlative
Correlative experiments are the least definitive because they cannot establish the relationship between
cause and effect. Here's a funny example from a letter to Nature:
A new parameter for sex education
Sir – There is concern in West Germany over the falling birth rate. The accompanying
graph1,2 might suggest a solution that every child
knows makes sense.
- Fachserie Gebiet und Bevölkerung (Statistisches Bundesamt, Kohlhammer,
Stuttgart, 1984).
- Bauer. S. & Thielcke, G. Die Vogelwarte 31, 183-191 (1982).
Reproduced without permission* from Sies, H. Nature (1988) 332:495.
|
Clearly fewer storks mean fewer babies. Many clinical studies fall into this category.
For example, people that
smoke have a higher risk for lung cancer. However, this does not prove that smoking
causes lung cancer.
Here's another example of a correlation: cars that have steering
wheels can steer. However, this does not establish that the steering wheel causes the
car to steer. Cars that can steer also have mirrors, so does that mean that mirrors are
also needed for steering?
Functional experiments are done to establish cause and effect
relationships. For example, if you remove a car's steering wheel, you can no longer
steer.
A plant's need for light provides a more scientific example.
For a loss of function experiment you could remove the light from the plant and it wouldn't
grow. For a gain of function experiment, you could shine more light on the plant and it
would grow more. Thus you may conclude that light causes a plant to grow.
4. Basic vs. Applied
Most research falls into one of two categories: basic and applied. Basic research asks
the question, "How does it work?" while applied research asks, "What can we do with it?"
Basic research is often considered a "higher" calling because if you want to find a cure
for cancer, you must first know how cancer works. However, this is not always the case.
Many cures were discovered and put to use long before their mechanisms were understood.
For example, the antibiotic penicillin was first used in World War II, but it wasn't
until decades later that researchers figured out that it kills bacteria by inhibiting
synthesis of their cell walls.
5. Ultimate vs. Proximate
Research can also be divided into ultimate and proximate categories. Take, for example,
the question "Why am I here?" An ultimate answer would involve my ancestors and the
origin of life on Earth. A proximate answer would describe how I walked into the room.
Humanity's fondness for sugar provides a more scientific example. An ultimate explanation
would be that the sugar present in ripe fruit was a signal to our ancestors that fruit was
ready to eat. A proximate explanation would be that the sugar binds to taste receptors
which stimulate a pleasure center in the brain.
The Ultimate is often more interesting to ponder, but the Proximate is definitely
easier to study.
6. Model Organisms
Because of things like ethics and the high cost of human experiments, the use of model
organisms is necessary.
You might not think we share much in common with yeast and wheat, but we
do. All living things are made up of cells, and the machinery operating inside each cell
is strikingly similar. Thus many important processes that occur in humans can be studied
much more easily in other organisms.
For example, all cells must fit about a meter of DNA into a space many times smaller than
the period at the end of this sentence. This is accomplished in part by winding their
DNA around proteins called histones. Shown below is a comparison of the amino acid*
sequences of the H3 Histone from humans, wheat, and baker's yeast. Because their sequences
are so similar, their structure and function are virtually identical.
*Since proteins are made up of chains of only 20 different amino acids, each amino acid is
represented by a letter of the alphabet in a standard code.
Human: MARTKQTARKSTGGKAPRKQLATKAARKSAPATGGVKKPHRYRPGTVAL
Wheat: MARTKQTARKSTGGKAPRKQLATKAARKSAPATGGVKKPHRFRPGTVAL
Yeast: MARTKQTARKSTGGKAPRKQLASKAARKSAPSTGGVKKPHRYKPGTVAL
Human: REIRRYQKSTELLIRKLPFQRLVREIAQDFKTDLRFQSSAVMALQEACE
Wheat: REIRKYQKSTELLIRKLPFQRLVREIAQDFKTDLRFQSSAVSALQEAAE
Yeast: REIRRFQKSTELLIRKLPFQRLVREIAQDFKTDLRFQSSAIGALQESVE
Human: ATLVGLFEDTNLCAIHAKRVTIMPKDIQLARRIRGERA
Wheat: AYLVGLFEDTNLCAIHAKRVTIMPKDIQLARRIRGERA
Yeast: AYLVSLFEDTNLAAIHAKRVTIQKKDIKLARRLRGE
Differences in amino acid sequence are highlighted in red
|
7. Genes: the central dogma of molecular biology
Aside from the influence of our environment, our genes are what make us who we are.
Your genes are what transformed a single fertilized egg into the 10 trillion cells of
your body. Each of your genes is encoded in the DNA of each cell, similar to how recipes
are recorded in a cookbook. Just as you would never make all of the recipes in a cookbook
all at once, each cell in your body only expresses a certain set of genes at once. That
is what makes the difference between a brain cell and a blood cell. When a cell expresses
a gene, it first makes an RNA copy of the gene, perhaps like you would make a working
photocopy of a recipe. Then the RNA recipe is read to make a protein. Proteins are the
molecules that do most of the work in living things, from carrying oxygen in your blood
to digesting your food.
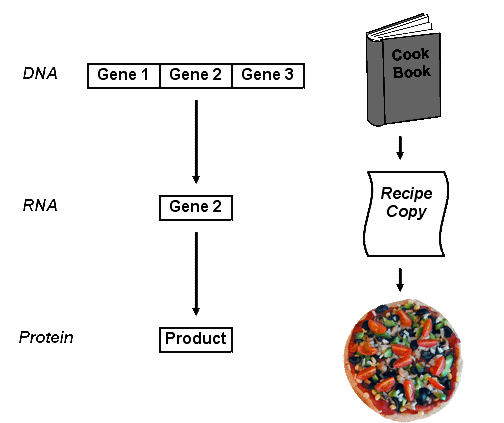
To figure out what a specific protein does, scientists usually try removing the gene that
codes for it (a loss of function experiment). Then they infer what the protein does by
studying what is wrong with the organism when the gene is missing.
8. Conclusion: Science is a way of looking
Science is merely a sophisticated way of looking at the world. Much of what scientists
see is not only logical, but also beautiful. Regardless of the origin of the Universe
and life, one must marvel at their beauty, complexity, and order. It is
amazing that our Universe follows logical rules that make sense. That in itself is
beautiful. What is even more amazing is that we, a part of our Universe, are capable
of understanding those rules. That understanding makes us unique - not even chimpanzees
know the size of the earth or how a cell divides.
The last few centuries have seen an incredible growth in our knowledge. The telescope
allowed us to see the worlds beyond, and the microscope allowed us to see the worlds within.
Some of the things we have seen have given us the power to heal and make life better (like
medicine). Unfortunately, other things we have seen have given us the power to inflict pain
and the loss of countless lives (like explosives). Science has made our lives safer and
healthier, but it has also made our conflicts more deadly and horrific. That is a risk that
scientists must take each time they look - they never know
what they're going to see.
Knowledge itself is neither good nor bad; it is we who make it so.
----------------
Acknowledgements
I would like to thank Caline for her ideas, Scott for help with the HTML, Seamus for his useful
critique, and the General Studies Honors Research Writing class of 2003.
*I followed the instructions on Nature's website to obtain permission to
reproduce this letter. Specifically, I emailed permissions@nature.com with
the pertinent information on June 26, 2005. To date I have received no response.
|